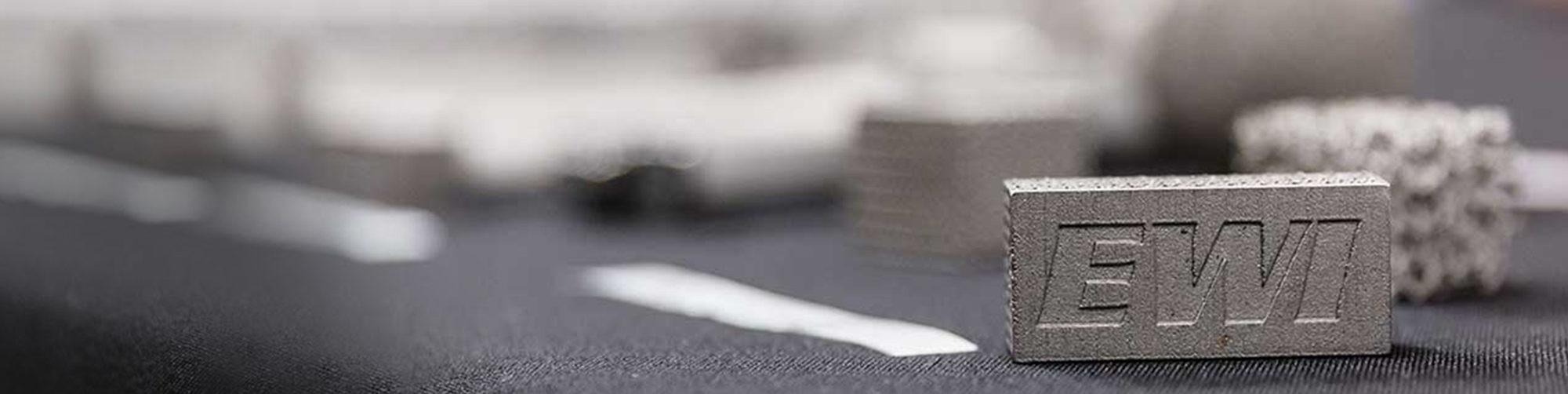
Non-metallic inclusions are residual products from de-oxidation, de-sulfidation and other chemical reactions which take place in the steel making process. Since these inclusions serve as heterogeneous nucleation sites during the solidification of liquid steel, they are often found at grain boundaries. Modern high-strength low-alloy (HSLA) steels are produced using thermo-mechanically controlled processing (TMCP) to roll steel slabs. Through the TMCP process, large inclusions can be broken down and re-distributed. The resultant distribution of smaller non-metallic inclusions limits grain growth at the normalizing temperature, yielding reduced grain sizes (around 25µm) and excellent mechanical properties.
It is well known that the thermal expansion coefficients of non-metallic inclusions differ from the surrounding steel matrix. Since the thermal expansion coefficients of sulfides, iron oxides, magnesium, manganese, and calcium oxides are higher than that of the steel,(1) they compress the steel to raise the local stress at a high temperature. On the contrary, other inclusions, such as silicates, calcium aluminates and alumina, can’t. However, they can generate a local tensile stress field after the steel is cooled. To control the thermal stress, steel makers have developed inclusion complexes consisting of sulfide coatings on silicates, calcium aluminates, and alumina to reduce the local tensile stress.(2)
While the engineered inclusion complexes effectively reduce localized tensile stress in HSLA steels, the welding of these materials becomes challenging due to the thermal behavior of non-metallic inclusions in the coarse grain heat-affected-zone (CGHAZ). Since CGHAZ is adjacent to the fusion line, grain boundaries at CGHAZ are highly mobile. Those mobile grain boundaries significantly promote grain growth. Some non-metallic inclusions, such as the eutectic Al2O3-CaO, have a melting-point around 1400oC, which is about the peak temperature in the CGHAZ adjacent to the fusion line. As a result, some inclusion complexes become molten or semi-molten during welding. Those molten inclusion complexes can coat the grain boundaries to increase the risk of de-cohesion in the steel matrix. As for a semi-melted inclusion complex, its volume can be shrinked at a high temperature, creating flaws in CGHAZ. Some non-metallic inclusions, such as calcium aluminates, are sharp-edged.(3) Flaws located near these sharp-edged sites may promote the secondary cracking under a tensile stress. As a result, they could significantly deteriorate fatigue strength, CTOD fracture toughness, and resistance to cold-cracking.
Diffusible hydrogen also plays an important role in premature failures in CGHAZ. When welding HSLA steels, weld consumable are often over-matched. The alloying elements used to strengthen these consumables usually raise the start temperature of the phase transformation from austenite to ferrite so that upon cooling, ferritic microstructures form in the weld before they form in the CGHAZ. This phase transformation near the fusion line significantly reduces the hydrogen solubility in the weld while the austenitic CGHAZ still has higher hydrogen solubility. As a result, diffusible hydrogen migrates into CGHAZ from the weld per Fick’s law of diffusion. The resultant high hydrogen concentration in the CGHAZ increases the risk of the premature failure after the cooling. Additionally, these non-metallic inclusions can absorb diffusible hydrogen to become hydrogen sources in steel.
Overall, to control the detrimental effects of non-metallic inclusions, the microstructure in CGHAZ needs to be carefully engineered to avoid a large volume fraction of hard phases, such as martensite/austenite (M/A) constituents. In addition, a welding consumable with a low hydrogen grade must be used to reduce the hydrogen diffusion flux from the weld metal to CGHAZ. Otherwise, localized embrittlement in CGHAZ may result in unsatisfactory mechanical properties.
References
- Brooksbank, D. abd Andrew, K.W., 1968, “Thermal expansion of some inclusions found in steels and relation to tessellated stresses”, Journal of Iron and Steel Institute, 206 (6), p 595-599.
- Mapelli, C., 2008 “Non-metallic inclusions and clean sttel”, La metallurgia italiana, p43-52.
- Kiessling, R., 1978, “Non-metallic inclusions in steel”, Parts I-IV. The Metals Society, ISBN 0-904357-18-X.
- DuPont, J., 2011, “Dilition in Fusion Welding” in ASM Handbook, Vol. 6A, Welding Fundamentals and Processes, ASM International.