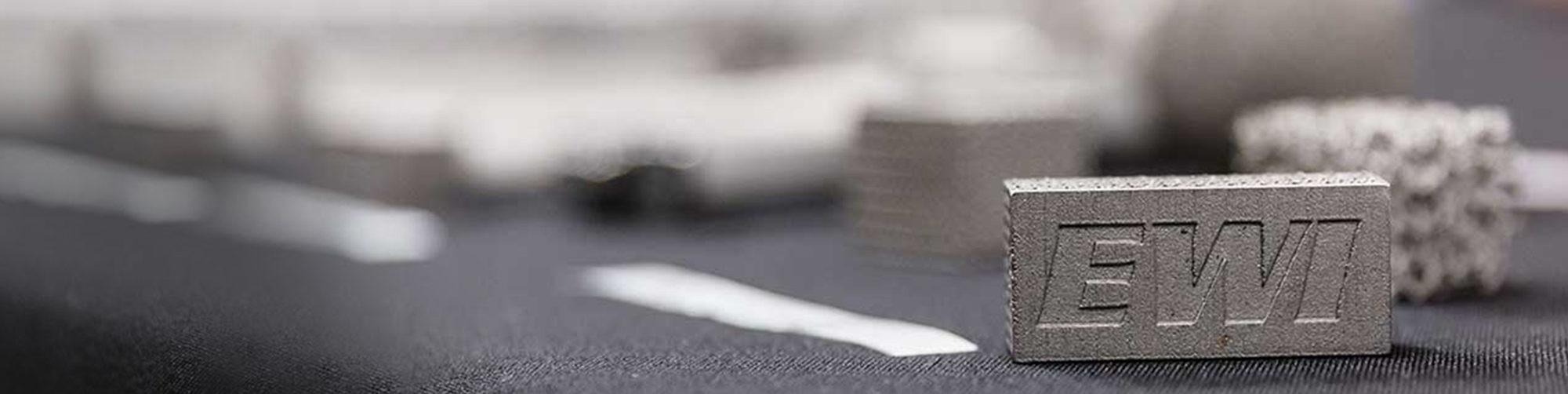
Reliable processing schedules for resistance spot welding have been readily available since at least the 1930’s. These welding schedules have been reproduced from handbook to handbook, and are currently available in the latest versions of the AWS recommended resistance welding practices (AWS C1.1M/C1.1:2000(R2006)). These practices are typically provided as a function of workpiece thickness. Generally, welding times, forces, and electrode sizes (not to mention welding currents) all scale monotonically with workpiece thickness. Analysis of these results shows that weld times vary as thickness to the 3/4 power . Alternately, weld forces vary , and face diameters as thickness to the 1/2 power. Existing resistance welding schedules have largely been developed material thicknesses ranging from roughly 0.5-mm up to about 3-mm. However, high precision applications have pushed resistance welding to significant thinner gauges (down to the 25-μm to 50-μm range). Also, higher power and force capability systems have facilitated production capable resistance welding up to a very large scale (EWI conducts resistance projection welding work to sizes up to 200-mm in diameter). In terms of the actual geometric (space) component of resistance welding, this range of applications represents effective physical scales varying by several orders of magnitude. It is worth revisiting the implication of such scales on the actual equipment and processing requirements for resistance welding. Within the conventional range of resistance spot welding applications, (0.5-mm up to about 3-mm) welding is effectively done with alternating current and straightforward pneumatic forcing systems. For typical welding times characteristic of this scale, there is sufficient thermal capacity such that there is little difference (in thermal response) between AC current and DC current. Also, weld times are sufficiently long such that the mechanical response of a number of pneumatic forcing system designs is appropriate for the process. These rules are not valid, however, as the scale of the application decreases. In this case, weld times quickly become short enough such that AC current appears as pulsation, or in the extreme a single pulse. Here, DC power is a necessity for proper weld development to occur. Also, these shorter times imply much faster response of the welding head is required to prevent force loss and subsequent expulsion. Alternately, welding at a large scale inevitably requires current pulsation to avoid localized overheating of the electrodes. In this case, AC and even frequency converter systems are attractive. Further, the long times associated with these larger scale applications often reduce mechanical follow-up requirements. The reduced requirement for mechanical follow-up then enables slower forcing systems (notably hydraulics) that also have the advantage of greater load capability in a smaller space. This discussion demonstrates that as the range of scales in resistance welding applications increases, greater care will be required in selecting the proper practice, power supply, and mechanical system to achieve consistent and reliable joints.